Practical Pulse Oximetry
Courtney Scales DipVN, NCert(Anaesth), RVN
Keith Simpson BVSc, MRCVS, MIET(Electronics) – Clinical Director
Joel Huey BA (Hons) – Content Editor
Introduction
Pulse oximeters are a widely available piece of patient monitoring equipment, used in veterinary anaesthesia. They are inexpensive, easy to use and provide non-invasive methods to gain real-time information on oxygen transport while providing an audible and visual pulse rate. The monitoring of pulse oximetry has been shown to reduce the risk of anaesthesia related morbidity and mortality (Brodbelt et al., 2008).
Oxygen Transport
Within the alveoli of the lungs, oxygen diffuses across the thin membrane walls and into the plasma of the capillaries that surround them. Most of the oxygen then binds to a protein called haemoglobin that is found within red blood cells, leaving only a small amount of oxygen dissolved in the plasma. The oxygenated blood is then carried from the pulmonary circulation to the left side of the heart, where it is pumped through the aorta, entering the systemic arterial circulation.
Haemoglobin
Each red blood cell carries millions of haemoglobin proteins and each of these proteins has four subunits that carry an oxygen molecule. Once all four subunits on the haemoglobin protein are carrying an oxygen molecule, it becomes ‘saturated’ and is referred to as oxyhaemoglobin, which is red in colour. Conversely, when haemoglobin is not saturated with oxygen, it is referred to as deoxyhaemoglobin and is blue in colour.
What is Pulse Oximetry telling us?
Before we discuss what, pulse oximetry is telling us, it is worthwhile to clarify some of the terminologies.
• We can measure how much of the haemoglobin in arterial blood is saturated with oxygen (sO2) via two different methods. The most common is with a pulse oximeter placed somewhere on the periphery, giving us a SpO2 reading. The other method is where an arterial blood sample is taken and analysed through a co-oximeter machine, giving us a SaO2 reading. Although SpO2 and SaO2 are often used interchangeably, they are not the same thing. As you can see, the SpO2 method of measurement is non-invasive and the SaO2 measurement requires an invasive technique.
• When we describe the “concentration” of a gas in a particular medium, usually liquid, we use the term partial pressure. We don’t actually measure what is in the blood, we measure the conditions that are needed to keep it there. For example, if we say the Partial Pressure of Arterial Oxygen (PaO2) is 80mmHg, then that is the result of that blood being in equilibrium with gaseous oxygen at 80mmHg.
The pulse oximeter machine uses a light absorption technique to determine how much of the haemoglobin is saturated with oxygen. We can compare the SpO2 against the Oxyhaemoglobin Dissociation Curve (ODC) and its relationship to the PaO2, allowing us to estimate the normal levels of oxygen in the blood available for cellular metabolism in the tissues. Alternatively, a more invasive and accurate way of obtaining this information is to take an arterial blood gas sample and analyse the PaO2 directly. It is the PaO2 that drives the diffusion of oxygen into the tissues, so think of SpO2 as the oxygen reservoir before it is released into the plasma. After the haemoglobin proteins are fully saturated, further increases in the PaO2 serve only to increase the content of dissolved oxygen in plasma, which is very small. This is described further in the section “Reliability and Limitations”.
There are some instances where there may be a left or right shift of the ODC, which can affect the binding and release of oxygen to the haemoglobin, such as changes from a metabolic or respiratory acidosis or alkalosis.
If the PaO2 cannot be measured with a blood gas analysis machine, it can be estimated by multiplying the Fraction of Inspired Oxygen (FiO2) by five. For example, room air is 21% oxygen and when multiplied by five, then we expect the PaO2 to be 80-100mmHg. In a healthy patient under general anaesthesia, breathing up to 100% oxygen, the PaO2 should be 400-500mmHg.
Hypoxaemia is defined as PaO2 less than 60mmHg, which corresponds to a SpO2 <90% (Schauvliege,2016), and you can see this represented in the ODC; if you make a line at 90% on the y-axis, it will meet the curve at 60mmHG on the x-axis. In a healthy patient breathing room air, the normal PaO2 is from 80-100mmHG at the alveolar level which correlates to a SpO2 of 95%-99%. We typically aim for a SpO2 in our patients that is >95%, however, for an anaesthetised patient that is breathing close to 100% oxygen (which should normally give us a PaO2 of 400-500mmHg), this would mean that a patient with a SpO2 <95% has a dangerously low amount of oxygen in their arterial blood. For there to be noticeable changes in the SpO2, the PaO2 must fall to a dangerously low level as a small change in the SpO2 percentage actually reflects a huge change in PaO2 for these patients.
One example of this is when we preoxygenate our patients prior to anaesthesia induction. After propofol administration and before being connected to a breathing system, desaturation (SpO2 <90%) occurs within 60 seconds in dogs that are breathing room air, compared to 300 seconds in those that are preoxygenated with 100% oxygen for 3 minutes (McNally et al., 2009). The PaO2 would have been rapidly decreasing in the preoxygenated patients, however it isn’t until the decreasing SpO2 is observed that all the “extra” dissolved oxygen in the blood has been “used”.
The cyanotic bluish mucous membrane colour which is associated with hypoxaemia is only visible when the SpO2 is <85%, therefore it shouldn’t be relied upon as an indicator of this.
Hypoxia
This is where there is the failure of normal tissue oxygenation, where those cells are deprived of oxygen. This may or may not be a result of hypoxaemia.
Hypoxaemia
This is where there is the failure of normal blood oxygenation and refers to the blood in circulation that is low in oxygen.
It is worth noting that a patient can be hypoxic and not hypoxaemic, which may occur in times of poor circulation (e.g., hypotension): the blood is well oxygenated but the circulation is insufficient to distribute it to the tissue.
Obtaining a SpO2 reading
The pulse oximeter obtains a reading by using a probe that emits two different LED lights, red light (660nm) and infrared light (950nm), across the tissue bed and into a receiver. The sequence of the LED lights is one goes on and then off, then the other and then they both turn off – this cycle repeats about 30 times a second. The machine then selectively reads the difference between the two light absorbances by the haemoglobin in pulsatile arterial blood flow:
• Deoxyhaemoglobin absorbs more red light allowing more infrared light to go through
• Oxyhaemoglobin absorbs more infrared light allowing more red light to go through
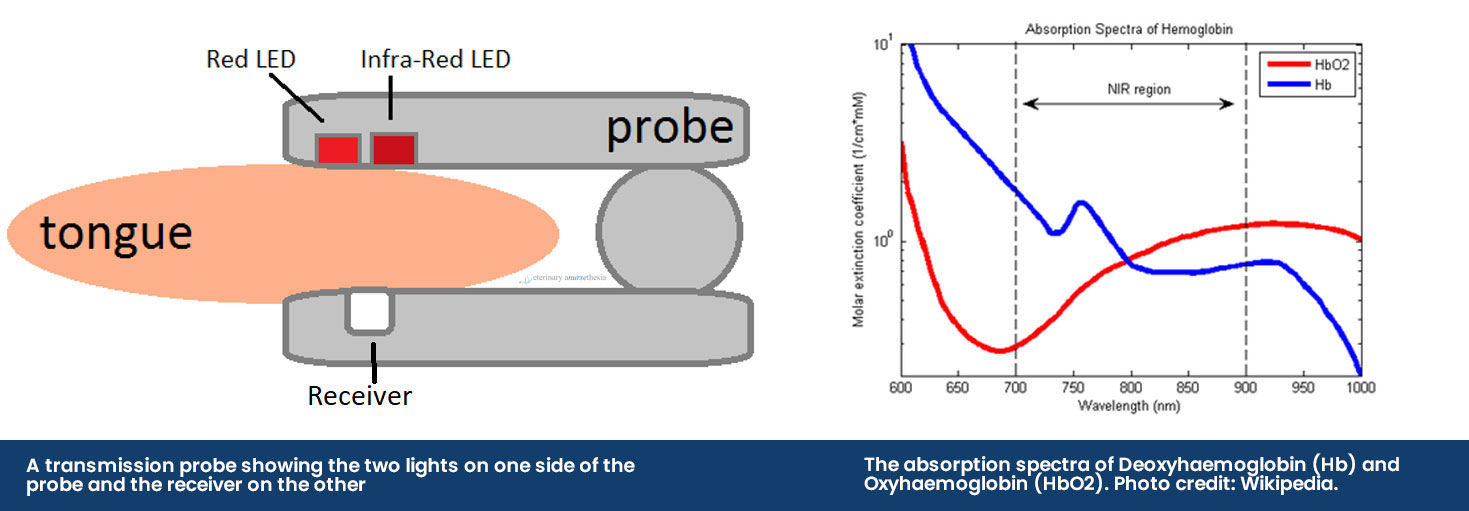
If a SpO2 reading is 97%, it means that 97% of the red blood cells that were read by the probe had haemoglobin proteins that were fully saturated with oxygen, and 3% were not.
The placement of the probe should be on pigment-free tissue where adequate contact can be made. This includes mucous membranes like the tongue and lip, the prepuce or vulva, the pinna or down the ear canal and the webbing between the toes. If the patient has dark or thick fur, readings may be difficult to obtain or they may be inaccurate.
There are two probes used to obtain pulse oximetry readings, the transmission probe and the reflectance probe.
The Transmission Probe
The transmission probe is the most commonly used in practice. The clip design holds a thin piece of tissue between it and emits infrared and red light from one side of the clip to a receiver on the other side. While these are easy to use, they can be prone to false errors and poor reliability as it compresses the capillaries, reducing the blood flow to be read.
The receiver side of the probe should make a seal against the fleshy mucous membrane to stop any light interference as shown below.
The Reflectance Probe
A reflectance probe is flat and has the infrared and red light on the same side as the receiver. This type of probe is taped onto an area of a patient, including a shaved area under the tail or it can be wedged down the ear canal. In human medicine, they are placed on the forehead in adults or on the foot in babies. This probe prevents the compression of the capillaries, but it can take practice to place and secure.
Another type of reflectance probe is a rectal probe. This provides accurate SpO2 readings providing there is contact with the rectal mucosa and not faeces.
Reliability and Limitations
We typically believe the reading if it is what we want it to be, such as a reading that is 95% or above, and we often dismiss readings if they tell us something we do not expect or believe to be true.
When validating a SpO2 reading, the accompanying plethysmograph should have a strong waveform that mimics an arterial blood pressure trace and the heart rate provided should also match the rate when auscultated. Some machines will be able to guide us as to if the reading is trustworthy or not. In the Lightning Multiparameter, signal strengths are graded as either A or B – where A is reliable, and B is less reliable, and the probe position should be checked.
When analysing the plethysmograph waveform, the upward stroke represents systole (the heart contracting) and the downwards wave represents diastole (when the heart is filling before the next beat). A dicrotic notch may be seen on the downwards stroke when the aortic valve closes and the distended aorta contracts, creating a brief change in pressure in the arterial circulation. Both the waveform and dicrotic notch can be interpreted alongside other parameters to gain information on the patient's cardiovascular status. A high dicrotic notch indicates vasoconstriction whereas a low dicrotic notch indicates vasodilation. The authors have found that when the plethysmograph has strongly identifiable waveforms, the SpO2 is usually reliable.
Despite a normal waveform, there are some instances where a normal SpO2 reading can be obtained, however, the patient has a poor oxygen-carrying capacity. This can occur in anaemic patients or those with dysfunctional haemoglobins.
Anaemia
Pulse oximetry obtains a reading based on the red blood cells present in the capillary bed as they pass the probe sensor, at that specific moment in time. A limitation in SpO2 readings occurs in anaemic animals as there are fewer red blood cells available and even if they are all fully saturated giving a normal reading, there is still a reduced oxygen-carrying capacity overall because there is less haemoglobin. Haemoglobin is estimated as ⅓ of the packed red blood cell volume (the haematocrit) which is normally 12.1-20.3g/dL in dogs and 9.3-15.9g/dL in cats (Klaassen, 1999).
Further to add, even if both the SpO2 and PaO2 are normal, it does not always indicate that the Oxygen Content of Arterial Blood (CaO2) is normal. To fully assess the oxygen-carrying ability of the patient, the haemoglobin (Hb) levels must be measured. The CaO2 calculation measures the bound (the SpO2) and unbound oxygen (the PaO2), just not one or the other. It can be calculated as follows:
CaO2 (mL/dL) = (1.36 × [Hb] × % saturation) + (PaO2 × 0.003)
1g of haemoglobin carried 1.36ml of oxygen and 0.003ml is the amount of dissolved oxygen in 100ml of blood.
For example, in a healthy canine patient breathing room air, that has a haemoglobin level of 15g/dL with a SpO2 of 95%, the CaO2 would be:
(1.36 × 15g/dL × 0.95) + (90mmHg × 0.003) = 19.65mL/dL
In a healthy canine patient breathing 100% oxygen under anaesthesia, that has a haemoglobin level of 15g/dL with an SpO2 of 100%, the CaO2 would be:
(1.36 × 15g/dL × 1) + (450mmHg × 0.003) = 21.75mL/dL
However, in an anaemic canine patient breathing 100% oxygen under anaesthesia, that has a haemoglobin level of 9g/dL with a SpO2 of 99%, the CaO2 would be:
(1.36 × 9g/dL × 0.99) + (450mmHg × 0.003) = 13.47mL/dL
The point of these examples is to show that the high saturation of the red blood cells and a high PaO2 do not always indicate normal oxygen content for that patient.
Dysfunctional Haemoglobin
Another reason for a patient to have hypoxaemia due to low CaO2 is due to dysfunctional haemoglobins. These haemoglobins do not carry oxygen:
• Carboxyhaemoglobin – this occurs when a patient is exposed to carbon monoxide (e.g. house fire), which has a higher binding affinity to haemoglobin than oxygen. Carboxyhaemoglobin provides falsely high readings even when the true oxygen saturation is low.
• Methaemoglobin – this is where the iron in the haemoglobin is oxidised from the ferrous (Fe2+) to the ferric (Fe3+) state and can no longer carry oxygen, lowering the SpO2 to 80-85%. It may be congenital or could occur in patients with paracetamol toxicity. Congenital methaemoglobinaemia has been reported in Pomeranian dogs (Shino et al., 2018).
In veterinary medicine, either of these two situations that cause dysfunctional haemoglobins is quite rare and they shouldn’t be a factor in troubleshooting erroneous readings, unless the clinical history is appropriate.
Hypoventilation
Pulse oximetry provides information on oxygen transport, not oxygen delivery to the tissue. Oxygen delivery is determined by the cardiac output and available haemoglobin. Additionally, it does not provide information on ventilation, where a hypoventilating patient under anaesthesia can have a normal SpO2, but a dangerously high level of carbon dioxide (CO2).
Troubleshooting abnormal readings
There may be certain situations in a healthy patient receiving a high FiO2 where the reading is erroneously low, which is more likely than an erroneously high reading (Haskins, 2015).
An erroneously low reading may occur for the following reasons:
- The administration of an alpha-2 agonist (e.g., medetomidine) causes peripheral
vasoconstriction, resulting in limited pulsatile blood flow available to obtain a reading. It usually occurs in the early stages of premedication and will eventually wane.
- If the patient is vasoconstricted, then it is more difficult for the probe to read pulsatile blood flow within the vessel. This may occur in hypothermic patients and actively warming the patient can improve blood flow to the periphery.
- Hypotension can reduce blood flow to the capillary bed and readings may be difficult to obtain. Measures to correct the blood pressure should be taken.
- Optical interference from room light can reduce the ability of the probe’s red and infrared light to differentiate the oxyhaemoglobin from deoxyhaemoglobin. The saturation level usually reduces to around 80%. Covering the probe with a drape or blanket helps to block out the room light, as does ensuring the receiver side of the probe is sitting snug against the tissue.
- Some transmission probes may have a tight clip that compresses and pushes the blood from the capillary bed, which is commonly seen when the tissue is thin, like little cat tongues. The probe can have its clamp loosened, wedged open (a syringe cap helps) or taped “open”. The use of a swab placed between the probe can help to align the sides of the probe and reduce the compression of the clip, but if the swab is wet it may make the tongue cold and cause vasoconstriction.
- The tissue where the probe is placed should be pigment and fur-free; the tongue, lip, prepuce,
vulva, pinna, or toe webbing can be used. If the patient has pigmented skin (e.g., a dark coloured tongue on a Chow Chow), then this can interfere with the light absorption techniques the pulse oximeter uses.
Insert Picture: A darkly pigmented tongue on a Chow Chow.
- Regional circulatory failure can occur if the SpO2 probe is on the same limb or extremity as a non-invasive blood pressure cuff, which is periodically inflated to occlude blood flow.
- In conscious animals, patient movement makes it difficult for the pulse oximetry probe to sense pulsatile arterial blood flow and a reading may not be provided at all.
- Pulse oximetry reads pulsatile arterial blood flow, however there are some instances where there is venous congestion in various organs which can lead to venous pulsation. These readings will be artificially lower as the venous blood has less oxygen in it, so this should be kept in mind when monitoring patients with left-sided heart failure or hepatic congestion.
Why might a patient become hypoxaemic?
Hypoxaemia can occur as a result of reduced oxygen delivery to the patient or due to the patient’s disease process that impairs the diffusion of the oxygen into the alveoli or the ability to carry the oxygen. Hypoxaemia during anaesthesia may occur for the following reasons:
- A low FiO2 is being delivered to the patient. This may be due to an insufficient fresh gas flow supply, a breathing system that is not correctly assembled, a kinked or long endotracheal tube, an exhausted oxygen supply or with the use of nitrous oxide.
- The patient may hypoventilate due to the respiratory depressive side effects that are caused by the anaesthesia drugs administered. Assessing and potentially decreasing the anaesthetic depth, changing patient positioning or supplementing breaths through Positive Pressure Ventilation should also be considered.
- Pulmonary disease can cause ventilation/perfusion (V/Q) mismatch or atelectasis. This is when there is a problem with how oxygen diffuses from the alveoli into the capillaries that surround them. In cases of V/Q mismatch, not all alveoli are being perfused or not all perfused alveoli are being ventilated. It can occur in cases of atelectasis, pneumonia, airway disease and fluid overload.
Using Pulse Oximetry in the Pre and Post Anaesthetic Period
Pulse oximetry is widely used in the peri-anaesthetic period, but it can also be used in the pre- and post-anaesthesia period.
The Pre-Anaesthesia Period
A SpO2 reading prior to the anaesthesia can help guide the expected value in the recovery period. For example, brachycephalic breeds are often chronically hypoxic so knowing “their normal” SpO2 prior to anaesthesia can manage expectations postoperatively.
The Post-Anaesthesia Period
If the patient is experiencing a delayed recovery, consider taking a SpO2 reading and providing supplemental oxygen. A patient may become hypoxaemic in the recovery period for several reasons, including:
- Hypoventilation from residual respiratory side effects of anaesthesia drugs once the patient is transitioned from a high FiO2 to room air, hypothermia or in patients that have increased intracranial pressure.
- Airway obstruction or airway disease can impact the delivery of oxygen to the patient’s lungs and could occur in brachycephalic breeds, cats suffering from feline asthma or patients with Acute Respiratory Distress Syndrome (ARDS).
- In patients with a diffusion impairment or ventilation/perfusion (V/Q) mismatch.
- Atelectasis, or the collapse of parts of the lung, commonly occurs under anaesthesia because of patient positioning and because of the delivery of a high concentration of oxygen under anaesthesia. It is usually self-limiting in the healthy patient and reverses after several hours during recovery.
How can the hypoxaemic patient be supported during the pre- and post-anaesthesia phase?
- If you have a conscious patient that you believe is truly hypoxaemic based on their low SpO2 reading, support can be offered by providing oxygen supplementation to increase the FiO2. This may be through flow-by, an oxygen mask or cage, or nasal prongs.
- If any anaesthesia drugs were administered that cause respiratory depression, these can be antagonised. Care must be taken that analgesia provided by opioids is not removed by antagonism, leaving the patient in pain. Positioning the patient in sternal recumbency can also be beneficial.
Key points to take away
Although pulse oximetry monitoring is prevalent in practice, the limitations of the information it provides in patients under anaesthesia receiving high concentrations of oxygen should be understood. Some key points include:
- A normal SpO2 reading does not always indicate adequate oxygen delivery to the tissue
- For there to be noticeable changes in the SpO2, the PaO2 must fall to a dangerously low level
- It provides an audible and visual pulse rate to the anaesthetist and theatre team
Burtons supply a wide range of pulse oximeters. From basic handheld options to complete multi-parameters with additional modules that can be added later to expand your monitors capabilities.
The Burtons Pulse Oximeter is your most basic option. Intended for use on dogs, cats and similar sized patients, the durable, compact and lightweight monitor is ideal for continuous monitoring of oxygen saturation (SpO2). The Burtons PM-60 is another compact handheld option, offering the additional benefit of a spot-check mode.
The M880B offers a compact handheld monitor that can be used as a capnograph and pulse oximeter, measuring CO2 and SPO2 signals in patients. Boasting a 4.3” auto-rotating touchscreen for ultra-clear readings, and a battery life of up to 18 hours, this monitor is ideal for accurate readings in any environment.
The NEW Suntech Vet30 & Vet30E can also be used as a pulse Oximeter. The Suntech range has been designed for BP measurement, but the more advanced options offer pulse oximetry and temperature measurement on top of this. Although still portable, the Suntechs are heavier and not as handheld as the previous monitors, these are more designed to be robust, with a rubber protective case and a flat base for sturdy positioning. The Suntech VET30E is suitable for large animals.
For a handheld monitor that offers even more measurement capabilities, we look to the PetMAP Graphic + SpO2. This lightweight, portable monitor is capable of measuring systolic and diastolic BP, mean arterial pressure, heart rate, temperature and SpO2. Again, boasting a clear touchscreen, you can easily interact with the PetMAP Graphic. Data can also be saved on an SD memory card via an integrated memory card slot.
Our larger multi-parameter monitors offer a more accurate way of measuring SpO2. Providing all required data shown on a clear screen so that all the results can be interpreted together. Some monitors even provide extras such as signal strength ratings and recognitions. As mentioned above, the Lightning Multi-Parameter Monitor offers you a signal strength reading of either A (good) or B (could be better), allowing you to adjust the probe position and try to get the most accurate reading possible. This is just one of many extra features of the Lightning Multi-Parameter Monitor. You can build this monitor based on your practice needs, adding the exact modules and extras that you require.
References
Brodbelt, D., Blissitt, K., Hammond, R., Neath, P., Young, L., Pfeiffer, D. and Wood, J. (2008). The risk of death: the Confidential Enquiry into Perioperative Small Animal Fatalities. Veterinary Anaesthesia and Analgesia, 35(5), pp.365-373.
Haskins, S. (2015). Monitoring Anesthetized Patients. In: K. Grimm, L. Lamont, W. Tranquilli, S. Greene and S. Robertson, ed., Veterinary Anesthesia and Analgesia, 5th ed. John Wiley & Sons, p.104.
Klaassen, J. (1999). Reference Values in Veterinary Medicine. Laboratory Medicine, 30(3), pp.194-197.
McNally, E., Robertson, S. and Pablo, L. (2009). Comparison of time to desaturation between preoxygenated and nonpreoxygenated dogs following sedation with acepromazine maleate and morphine and induction of anesthesia with propofol. American Journal of Veterinary Research, 70(11), pp.1333-1338
Schauvliege, S. (2016). Patient Monitoring and Monitoring Equipment. In: T. Duke-Novakovski, M. de Vries and C. Seymour, ed., BSAVA Manual of Canine and Feline Anaesthesia and Analgesia., 3rd ed. Gloucester: John Wiley & Sons, p.83
Shino, H., Otsuka-Yamasaki, Y., Sato, T., Ooi, K., Inanami, O., Sato, R. and Yamasaki, M., 2018. Familial Congenital Methemoglobinemia in Pomeranian Dogs Caused by a Missense Variant in the NADH-Cytochrome B5 Reductase Gene. Journal of Veterinary Internal Medicine, 32(1), pp.165-171.
Brodbelt, D., Blissitt, K., Hammond, R., Neath, P., Young, L., Pfeiffer, D. and Wood, J. (2008). The risk of death: the Confidential Enquiry into Perioperative Small Animal Fatalities. Veterinary Anaesthesia and Analgesia, 35(5), pp.365-373.
Haskins, S. (2015). Monitoring Anesthetized Patients. In: K. Grimm, L. Lamont, W. Tranquilli, S. Greene and S. Robertson, ed., Veterinary Anesthesia and Analgesia, 5th ed. John Wiley & Sons, p.104.
Klaassen, J. (1999). Reference Values in Veterinary Medicine. Laboratory Medicine, 30(3), pp.194-197.
McNally, E., Robertson, S. and Pablo, L. (2009). Comparison of time to desaturation between preoxygenated and nonpreoxygenated dogs following sedation with acepromazine maleate and morphine and induction of anesthesia with propofol. American Journal of Veterinary Research, 70(11), pp.1333-1338
Schauvliege, S. (2016). Patient Monitoring and Monitoring Equipment. In: T. Duke-Novakovski, M. de Vries and C. Seymour, ed., BSAVA Manual of Canine and Feline Anaesthesia and Analgesia., 3rd ed. Gloucester: John Wiley & Sons, p.83
Shino, H., Otsuka-Yamasaki, Y., Sato, T., Ooi, K., Inanami, O., Sato, R. and Yamasaki, M., 2018. Familial Congenital Methemoglobinemia in Pomeranian Dogs Caused by a Missense Variant in the NADH-Cytochrome B5 Reductase Gene. Journal of Veterinary Internal Medicine, 32(1), pp.165-171.